Computational Modeling
The Rising Sea Level
The Clock is Ticking on the Doomsday Glacier
Computational modeling sheds light on the Thwaites (aka. ‘Doomsday’) Glacier.
By Celia Konowe
Front of Thwaites Glacier.
© ITGCAlong the West Antarctic Ice Sheet (WAIS), measuring between 800 and 1200 meters deep at its grounding point, is the Thwaites Glacier. About 120 km (~80 mi) wide, it is Earth’s widest glacier and its melting currently contributes to around 4% of all global sea rise, as reported by the International Thwaites Glacier Collaboration (ITGC), a collaboration between the U.S. National Science Foundation and UK Natural Environment Research Council. Thwaites’ nickname as the Doomsday Glacier is apt; if the glacier were to collapse completely, global sea levels would rise by more than two feet (65 cm, 25 in). In the face of a ticking clock, scientists at ITGC are racing to better understand cryosphere processes and minimize uncertainty in glacial melt models.
Change at Thwaites is driven by interactions between the glacier and a warming atmosphere and ocean, marking the largest ice-ocean shifts occurring in Antarctica. Annually, Thwaites loses about 50 billion tons of ice more than it receives in snowfall according to ITGC, accumulating in a net loss of more than 100 billion tons since 2000. ITGC consists of eight projects to better understand the glacier: four focus on natural processes, two explore the historical context of this part of WAIS; and two utilize modeling techniques. Three scientists, each representing a different project, joined the discussion to shed light on their work and the role of modeling in Thwaites’ future.
-
Dr. Richard Alley is the Evan Pugh University Professor of Geosciences and Associate of the Earth and Environmental Systems Institute at the Pennsylvania State University and is a member of GHOST (Geophysical Habitat of Subglacial Thwaites).
-
Dr. Mathieu Morlighem is a glaciologist and the Evans Family Distinguished Professor of Earth Sciences at Dartmouth University and is a member of PROPHET (Processes, drivers, Prediction: modeling the History and Evolution of Thwaites).
-
Dr. Jeremy Bassis is a professor in the Department of Climate and Space Sciences and Engineering at the University of Michigan and a member of DOMINOS (Disintegration of Marine Ice-sheets Using Novel Optimized Simulations).
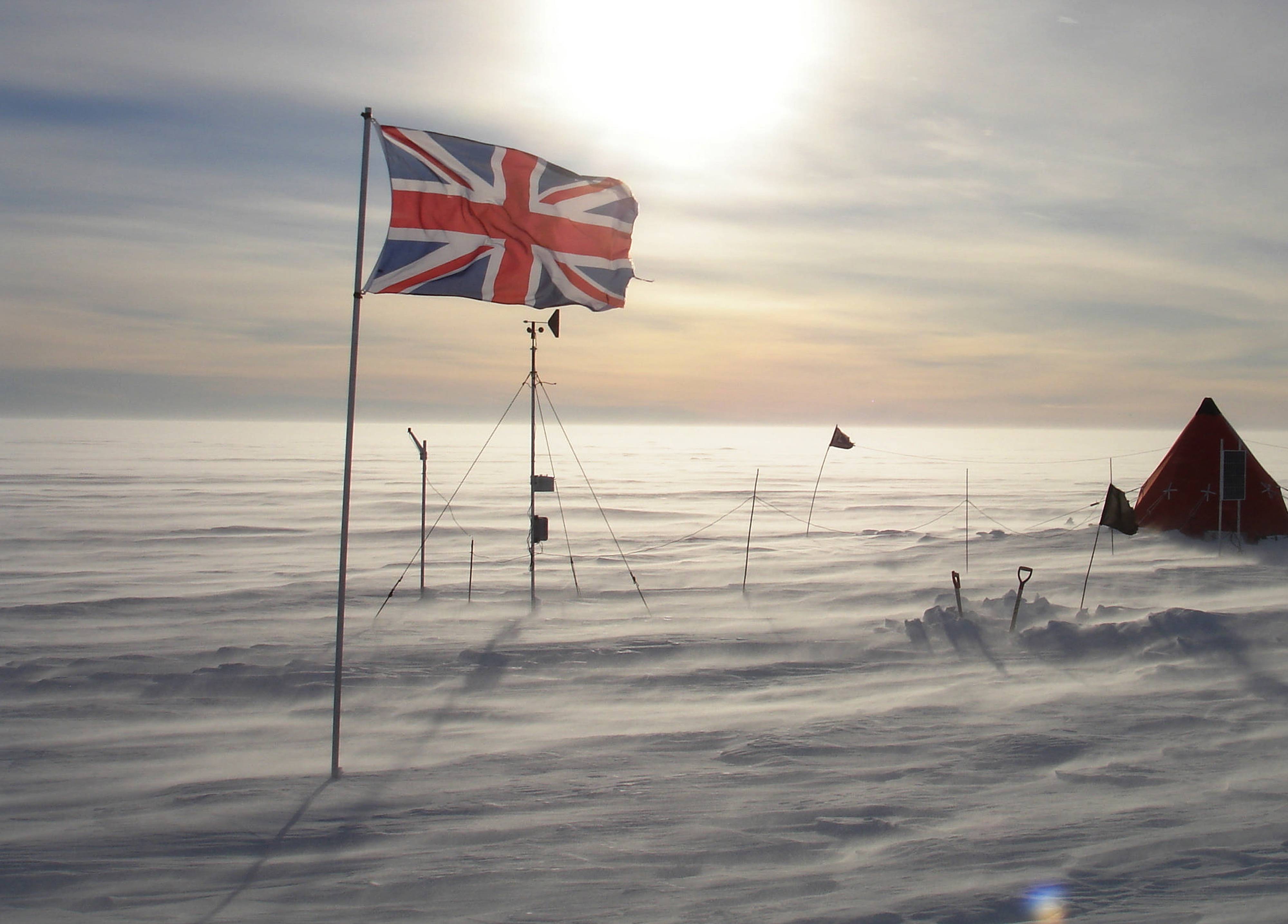
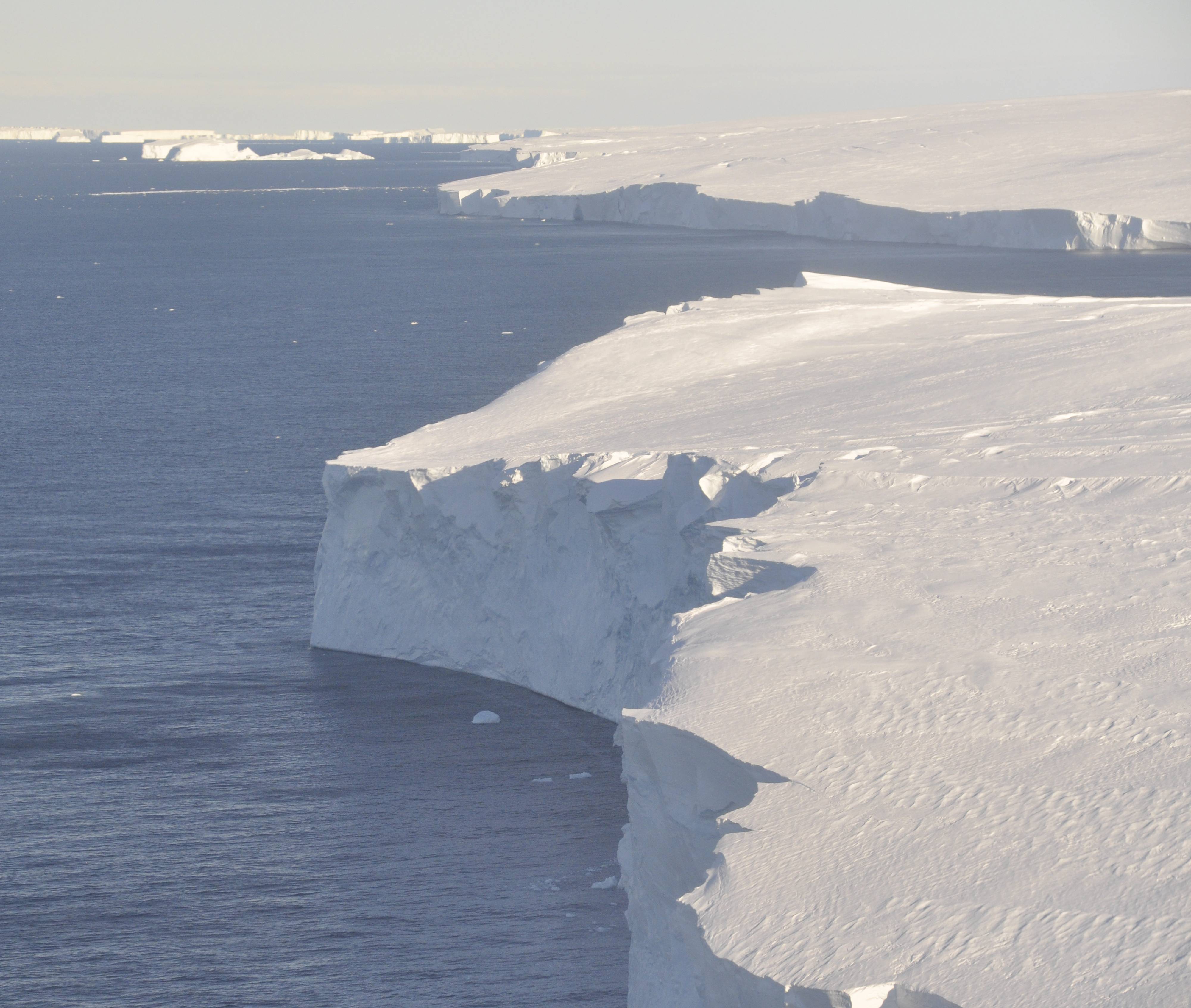
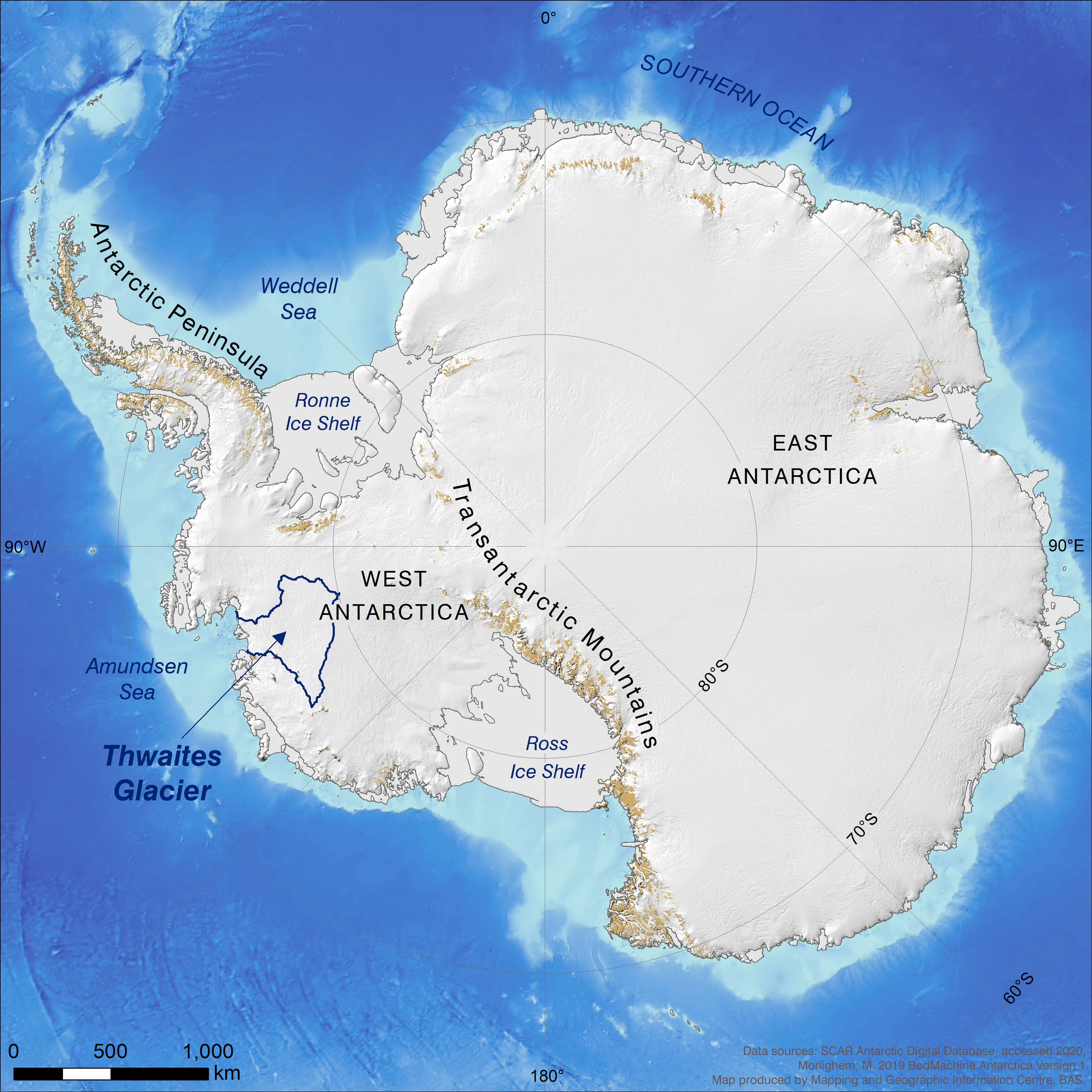
What role(s) do your respective projects play in understanding the future of Thwaites and the ice-ocean relationship?
Alley: GHOST examines the rheology and topography of the bed, which affect how quickly Thwaites retreats and contributes to sea-level rise. I mostly work to identify active physical processes and field work that can characterize the activity of these processes, build simple physical models of those processes, assess their importance, and help incorporate them in complex models.
Morlighem: I'm an ice sheet modeler. I develop new computer models to try to better predict how the ice sheets are going to respond with climate change. I look at specific glaciers like Thwaites, but also the whole ice sheet. One goal of PROPHET is to use these new tools and our new understanding of ice dynamics and to look into the future of Thwaites as an ice ocean coupled system. The role of PROPHET is not to go out in the field, but to use the data that have been collected by other teams to synthesize and see how that work ITGC is affecting projections.
Bassis: My work involves detailed process modeling of how ice flows and breaks. The modeling community is skilled at modeling the longer-term flow of ice sheets and glaciers, but one of the big challenges is that ice also breaks. Ice that has fractures in it can flow much faster than intact ice, but more importantly, cracks in the ice result in the detachment of icebergs. Much of the work that we do in the DOMINOS project seeks to better understand the interplay between flow and fracture and to better determine how iceberg calving will impact projections of sea level rise.
At the time of writing, some members of GHOST are on their way home from an expedition on Thwaites. Richard, can you provide an update on the data gathered?
Alley: The GHOST team had a wonderful expedition, arguably the most ambitious coordinated glaciological geophysical traverse ever. The collection of geophysical data that was completed will give an unprecedented view of the ice and its substrate.
Because of logistical difficulties, not all work could be completed, but the team planned extensive active seismic experiments, using both small explosions and a repeating mechanical “thumper” to generate sound waves, which were recorded on geophones. Furthermore, they planned for extensive monitoring of natural seismicity from formation of crevasses and from motion of debris-bearing ice over bedrock using a variety of radars.
PROPHET and DOMINOS employ varying modeling techniques with different inputs and outputs. Can you expand?
Morlighem: What we do is called inverse modeling. We assume something about the bed, like, "Oh, it's very slippery," or, "No, it's very sticky." The model will tell you how fast the ice is flowing, but then we can compare it to what we observed at the surface ice from satellites, and we can correct for our basal conditions based on the misfits between the model and observations. There are so many parameters that we don't have good measurements of; inverse modeling helps us get to a state that's consistent with today.
The three models that PROPHET is using are ISSM, Úa, and STREAMICE, which is part of MITgmc. ISSM is 2 and 3D, whereas STREAMICE and Úa are both two-dimensional, so we can integrate the three to look at the glacier from above in depth. They’re all based on similar approaches—maybe a slightly different way of describing ice flow, but if we end up with similar results, it shows that the conclusions are robust.
Bassis: We use a range of models that operate on different spatial and temporal scales. At the largest scale, we use the BISICLES model to simulate the flow of ice over hundreds of kilometers. This model treats the ice like a viscous fluid, but the model doesn't resolve cracks or icebergs. We then use a range of models that have meter-scale resolution and cover regions that are 10s of kilometers in scale. These start to resolve how ice fails and flows but are only able to simulate this behavior over a smaller region. Both models are "continuum" models, treating ice like a fluid. Finally, the HiDEM discrete element model treats ice like it’s glued together by elastic bonds. This model only resolves the short-time scale of brittle fracture of ice over minutes but is considered "high-fidelity" because it can fully resolve the fracture process.
Artificial intelligence is a growing field, for better or worse, across countless disciplines. Does it yet play an impactful role in cryosphere assessment and modeling?
Morlighem: AI I think is great for when we have so much data, like images that are collected every day and it's too difficult for humans to analyze all of them. In terms of models and physics, it's still new—I'm working on it myself. We use PINNs, or physics informed neural networks, to expose the AI not only to the data, but also to the physics.
The question really is, are they going to be better than classic traditional models? We haven't seen anything that traditional models cannot do and cannot do faster because training a neural network is very time-consuming. With Thwaites, you can do that with a traditional model in a few minutes. With a neural network, you need several hours. I think it has potential; we just haven't found that potential yet through that application.
Thwaites may be nicknamed the Doomsday Glacier, but is the future all doom and gloom? What gaps remain?
Alley: The progress from the field work, modeling, and remote sensing have been spectacular, but much remains to be done. The future of the ice sheet, and thus of sea level, is a coupled problem that depends heavily on behavior in the Southern Ocean around Antarctica as well as behavior of the ice and its bed, with the ocean behavior linked to global trends. Most of the major prognostic ocean-atmosphere models being used to study global climate change still treat Antarctica as a large, white mountain. Merging the field data, the local and regional ice-flow models, and the global ocean-atmosphere models is a major challenge.
Morlighem: It wasn’t until the early 2000s that the science community realized they didn’t have the right tools and models to replicate what they were seeing in ice sheet melt. Unexpected dramatic shifts, like the Larsen B fracture in 2002 helped realize this. Ice sheet modeling has been a slow process because it's so new, especially compared to ocean or atmospheric modeling where we've been making weather forecasts for a long time. Our goal is to reduce uncertainty by focusing our attention on one single glacier, collecting data, and better understanding processes and how to initialize models. The uncertainty right now is just too high for policy makers to make decisions now. I hope our work with PROPHET helps at a larger scale get that uncertainty down.
Bassis: Models aren't crystal balls, but they do tell us about what might happen in the future. We try to make those projections a little bit more realistic. Sometimes we can use those improvements to tell if some of the worst-case scenarios for sea level rise are more or less likely. The reality, however, is that the choices we make to stop burning fossil fuels and to adapt to climate change and sea level rise has a much greater impact on what the future holds than any of the uncertainties that exist in our models and projections.
With a recent expedition under their belt and a ticking clock, ITGC has their work cut out. Computational models, varying across projects, will assist in assessing the ice sheet and maintain robustness. While the Doomsday Glacier is not alone in having a gloomy future, it remains a key player in ice-ocean interactions. Global collaboration across scientific groups will reduce uncertainties, more accurately informing policy and mobilizing communities to make sustainable mitigation decisions in the face of mass glacial melt and sea level rise.
What is happening to the Thwaites Glacier?
Alley: Briefly, an ice sheet is a pile of old snow, squeezed to ice under the weight of more snow, and spreading under its own weight. In colder places (including most of Antarctica), cold air and water allow the ice flowing into the ocean to remain attached as a floating ice shelf.
Almost all ice shelves run aground locally on small bedrock highs or else flow past rock or ice walls, providing friction that slows the ice shelf and in turn slows flow of the non-floating ice into the ocean, keeping the ocean smaller and the ice sheet bigger than they otherwise would be.
Thwaites is thinning, and the zone where it enters the ocean is migrating toward the center of the ice sheet, in response to reduction in the “buttressing” from the friction holding back its ice shelf, primarily in response to warming of the ocean waters under the shelf, which mostly resulted from changes in the winds bringing in warmer waters.
Evidence from elsewhere and model understanding (including some of our work) show that beyond some threshold, ice shelves may break off entirely, producing the non-ice-shelf calving that is observed around much of Greenland and in parts of Antarctica. Whether this will happen at Thwaites, and whether the grounding zone will retreat far enough to reach the interior basins with their very deep beds, which could make very high calving cliffs that could break rapidly, remain deeply uncertain.
About the Author
Celia Konowe is from Reston, Va., and has a bachelor’s degree in environmental studies. She has study abroad experience in France and Ecuador. Currently, she is pursuing her master of environmental studies degree at Dalhousie University.